Swimmy the fish
Swimmy » Be A Learning Hero
Swimmy by Leo Lionni
Have you ever felt different? Where Swimmy lives, he is the only fish that isn’t red. He also swims faster than all of the other fish. Then Swimmy is the only fish to survive an attack from a bigger fish, leaving him all alone. He sets out on his own to find a new school of fish and along the way learns a lot about the ocean around him. You will be rooting for Swimmy as he uses his new knowledge to help his new friends. Your family will enjoy watching this small fish grow in big ways. Leo Lionni uses a variety of painting methods to create colorful images that will catch your child’s attention as you read about Swimmy’s adventures. Children will relate to Swimmy’s unique attributes and will learn that differences are often strengths!
Vocabulary
It's important to make sure that your child has an understanding of key words in the book. Talking about words while reading is a great way for your child to learn new words.
In this book, you might talk about these words:
- fierce (pg. 1)
- invisible (pg. 3)
- school (pg. 5)
You might use a question like:
In the book, what does the word fierce mean? How does the image help you understand the
word?
Key Ideas and Themes
In addition to words, it's important to talk about key ideas and themes and how they develop over the course of the book.
Here are some examples to get you started:
- 1
At the beginning, the book talks about how Swimmy is different from other fish. See if your child can name some of his differences.
- 2
Events leave Swimmy all alone. Talk about how he responds to his new situation.
- 3
After Swimmy finds the new school of fish, he says, “Let’s go and swim and play and see things!” What does this show you about his point of view of the things he saw on his journey?
- 4
Swimmy’s new friends fear big fish.
Talk about how he responds to their fear. How does the story come to an end?
- 5
Swimmy changes throughout the story. See if your child can point out ways he changed and parts of the story that show his changes. Talk about what can be learned from Swimmy’s transformation.
- 1
The illustrations in Swimmy support the ideas in the text and often add more details to the story. As you reread the story together, stop on each page and discuss the author’s choices in images. Think about the colors, shapes, and pictures he chose to use and ask, “How do the pictures add to the ideas in the text?”
Swimmy
None Swimmy explores the deep ocean and uses his imagination to help new friends. In a corner of the sea, Swimmy, the little black fish in a school of little red fish, is faster than his brothers and sisters.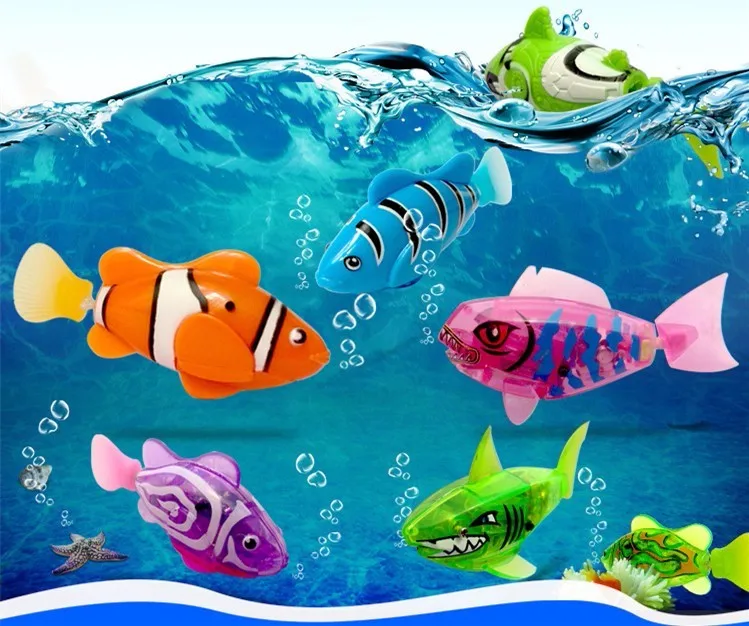
Classics
Share your favorite stories with your child. Enjoy classic bedtime stories from your childhood like Chicka Chicka Boom Boom, Chicken Little, Where the Wild Things Are, and Harold and the Purple Crayon.
view all
Chicka Chicka Boom Boom
Harry the Dirty Dog
Wheels on the Bus
Chicken Little
The Snowy Day
The Dot
Where the Wild Things Are
Duck on a Bike
Swimmy
Harold and the Purple Crayon
One membership, two learning apps for ages 2-8.
TRY IT FOR FREE
Full Text
In a corner of the sea there lived a happy school of little red fish. Only Swimmy was as black as a mussel shell, and he swam faster than his brothers and sisters. One bad day, a tuna, fierce and very hungry, was hunting for a juicy prey. Suddenly he saw the little fish playing in the sunny water. There were no rocks, no weeds to hide in, and when they saw the glittering teeth, they darted here and there. It was too late. In a few hungry gulps, the tuna swallowed all of them. And then, with his belly full he quietly swam away as if nothing had happened. But in the confusion, Swimmy had managed to escape. He swam away in the deep, wet world. He was scared, lonely, and very sad. Then things began to happen. A jellyfish, full of the colors of the rainbow, floated by. And behind a screen of weeds an object full of prickles lay in the sand. Out came a crab with claws like the beaks of birds. “Catch me!” said Swimmy.
“Catch me. Catch me if you can!” But a cuttlefish was pumping its way through the sea. And then it was night. Swimmy discovered a forest of seaweeds growing from sugar candy rocks. Large fish floated by, pulled by invisible threads. And out of tiny vases large ocean flowers unfolded at the touch. And what was that? An eel—so long that his tail was almost too far away to remember. There were tunnels in the rocks. Luminous fish flickered in the dark. The world was full of wonders, and Swimmy was happy again. Then, in the deep shade of weeds, Swimmy saw a school of little fish. They were just like his brothers and sisters. “Let’s go and play and see things!” he said excitedly. “We can’t,” said the little fish. “The big fish will eat us all.” “But you cannot live this way,” said Swimmy. “We must think of something.” Swimmy thought and thought. He thought of this, and he thought of that, and then he thought some more. And suddenly he said, “I have it!” “We’re going to swim together, each in his place.
” And when they had taken the shape of one large fish, Swimmy said, “I’ll be the eye.” They swam into the open water where the tunas hunted and they chased the big, bad fish away.
1
We take your child's unique passions
2
Add their current reading level
3
And create a personalized learn-to-read plan
4
That teaches them to read and love reading
TRY IT FOR FREE
Is swimming like a fish a useful technique for swimmers?
<
p style="text-align: justify;"> Introduction
In August 1998, we featured an article by Australian science journalist Daniel Drollett published in the British journal Science: ["Swim like a fish", see below ]. Ten years later, we believe the article is still relevant.
Daniil Drollett tries to answer the burning question, is there any other way to swim faster than simply increasing your mileage to improve your progress. Turetsky, Popov and many other great swimmers have shown that this is possible by reducing drag. Some people call this type of swimming “swimming like a fish” because fish, in particular, are nimble creatures and have optimized drag and thrust. Swimmers need to figure out how they can reduce their drag to develop the most efficient forward propulsion.
At first, the principle of "swimming like a fish" or "fish-like swimming" seems convincing. However, we are definitely not fish. If you understand the slogan word for word, then the idea is completely wrong. We cannot move our body and limbs like a fish. Instead, this slogan can help us navigate the water with ease, grace, and efficiency, as do fish and marine mammals.
<
p style="text-align: justify;"> The laws of hydrodynamics apply to everything that moves in water.
Another "model" that is often used is the "racing boat" model. These boats are long and narrow, which reduces drag. If we apply this model to swimming, then your body must be kept long, narrow, and flat so that drag is constantly minimal. The image of a racing boat will help visualize the streamlined body position and posture in the water.
<
p style="text-align: justify;">The third topic in the article, which will be covered by Turetsky below, is stroke frequency and stroke length.
This topic has caused a lot of controversy, mainly in the United States. Turetsky's ideas were adapted by Total Immersion for doing business. The founder of Total Immersion, Terry Laughlin, was accused of incompetence in competitive swimming.
To illustrate this controversy, we publish two letters to the editor of Swimming Technique, a US swimming magazine. In one of the publications, Terry Laughlin published an article entitled "Swim Like a Fish".
Gennady Turetsky
Alexander Popov
<
p style=”text-align: justify;”> Swimming technique: swim like a fish
Source: New Scientist, 1. August 1998, no. Gmünder
<
p style="text-align: justify;"> Forget about brute force
If you want to swim really fast, stop jerking, relax and feel the water. Olympic team coach Gennady Turetsky told Daniil Drollett how to overcome the opposition.
<
p style="text-align: justify;"> There is humiliation and there is real humiliation
While swimming in a local outdoor pool in Canberra, some guy blasted past me like a torpedo. I felt unwell. I actually swayed in the water when he swam by. But that feeling didn't last long once I learned that the torpedo was Alexander Popov, a two-time Olympic gold medalist and world record holder in the 100m freestyle. On the next track, the formidable shaved head of Popov's training partner, Michael Klim, gleamed, who swam 100 meters butterfly in the shortest possible time. I involuntarily climbed into the part of the pool where these elite athletes sometimes train for a change of scenery, from the indoor pool at the nearby Australian Institute of Sport.
Popov seemed to glide easily through the water, elbow bent and pointing up in the classic crawl position, long arms cutting into the water ahead with elegant ease. Unlike him, Klim brought down his arms in front, clearly straight, as soon as they emerged from the water, in his “windmill” crawl style. The style of each of the men is unique, but both are world leaders. And both are products of the extraordinary ideas of the same coach, Gennady Turetsky.
<
p style="text-align: justify;"> A flamboyant and sometimes controversial Turkish character, studies the movement of fish and writes physics equations on a blackboard in his poolside office to explain the principles of fluid dynamics. His brand of evidence-based training has done a lot to promote the idea that it's not brute strength that makes champion swimmers, but efficiency. Klim and Popov were taught to behave like a fish in water in order to "feel" the water and glide through it.
Now, a naturalized Australian, Turetsky is a product of the old Soviet system, where as many as eight scientists would watch the performance of the national team. He is a former champion swimmer and holds degrees in engineering and training in biomechanics, biochemistry, fluid mechanics, and sports physiology. He is known for using unusual props to get his ideas across: he once brought an inflated condom to the pool to show his fellow coaches the importance of keeping a rigid core while kicking. When air was released from the condom, it dangled listlessly in the water; when inflated, it glided over the surface from one light push.
Turkish swimmers pray for him. For example, it was Turetsky's idea for Klim to switch to the windmill style. "I've been straight up breaking personal records ever since he made me make this change," Klim later told me. Popov is even more enthusiastic: "He is the reason why I left Russia." Popov and Klim have the status of pop stars in Australia, while magazines and newspapers call the Turkish man who changed the top echelon of navigation in Australia. But amid all this hype, the question remains: how do Turetsky and his swimmers do it?
The answers, Turetsky says as he steps steadily along the side of the pool, lie partly in genetics and partly in technology. Elite swimmers are usually born with certain advantages, such as super efficient metabolism. In some long distance swimmers, for example, the cardiovascular system is able to deliver twice as much oxygen to starving muscle cells as in a young adult of average fitness, thus giving them an advantage even when entering the pool.
Olympic swimmers also tend to be tall with long limbs. On land, both Turkish swimmers are as long and lanky as the basketball players. Klim is 1.91 meters (6 ft 3 in), while Popov, at 1.97 m (6 ft 6 in), can touch the bottom of the deep side of the Canberra Basin and still keep his head above the water. This pair is made for swimming. Or as Turkish told them: “You have something given to you by God. You must develop it." Fair enough. But how?
<
p style="text-align: justify;"> There are two ways to swim faster, Turkish says: increase the force swimmers use to propel themselves through the water, or decrease the resistance of the water. Both approaches have their origins in technique, but he thinks the second approach is unquestionably the best.
To propel yourself through the water faster, you could, for example, increase your stroke rate. But there is a problem here, Turetsky says. You will soon be out of breath. He cites an excerpt from his favorite book, The Swimming of the Fish by zoologist John Wiedeler at the University of Groningen in the Netherlands, which says that energy consumption in water increases as the cube of stroke rate. In other words, doubling the speed at which you move your hands in water requires eight times as much energy.
Moreover, an increase in the frequency of strokes inevitably means shortening of their length , which is contrary to how most animals behave. When they want to move faster, they increase the distance they cover with each move. Turetsky shows video clips as proof: horses, Turetsky notes, accelerate, increasing the distance they cover with each stride without increasing the number of steps per second. Kangaroos do the same, jumping on their two legs. Turetsky believes that swimmers should do what animals do, stretching as far forward as possible to get the longest possible grip with each stroke. Popov's first gold medal at the Olympics 1992 years in Barcelona provided evidence to support this approach. When he defeated American swimmer Matt Biondi, Popov made only 33 strokes for 50 meters, against Biondi's 36.
So if increasing stroke rate isn't the solution, how about getting a stronger grip and pulling through the water? Until the 1980s, swimmers and their coaches focused on strength. They drew inspiration from mechanical models such as propellers and propellers. The typical swimmer had the shoulders of a Bulgarian weightlifter and the emphasis was on multi-kilometer training sessions, according to notorious coach Cecile Colwin, author of Swimming into the 21st Century. The science of biomechanics "was incorrectly focused on emulating the actions of mechanical propellers instead of mechanisms more like the natural flight and propulsion of fish," she wrote.
Turkish agrees with Colvin, for reasons based on physics. Fluid dynamics tells us that drag depends on shape and friction. For example, dolphins swim so fast because they are streamlined and because their skin is designed to reduce friction by stopping energy-absorbing vortices from forming around their bodies.
<
p style="text-align: justify;"> Create a wave
Humans don't have any of these benefits. But the real killer for swimmers - athletes - is the third type of resistance that occurs at the border between air and water - this is wave resistance. Movement on the surface of water inevitably creates waves. From a physical point of view, swimmers apply force to the body of water in front of them to lift themselves up against the force of gravity. This not only drains swimmers of energy, but also has a disproportionate effect the faster they swim.
The problem is that wave drag increases with the cube of any increase in swimming speed. And it gets even worse if the swimmer makes jerky or uneven movements, or jumps in the water or wobbles from side to side, because this wastes more energy, causing waves. Because of this, Turetsky believes that trying to increase speed by pushing yourself through the water with force is pointless beyond a certain point. “More propulsion will only cause higher waves, but not more speed,” he says.
If you can't control water, Turkish is convinced, then you'd better learn to avoid its inhibitory influence. For starters, reducing friction with water is essential. This is one of the reasons why Klim shaves his head. The shape or outline is also an important factor. For swimmers, this means getting into a streamlined position with tricks like pushing the head and chest down into the water, and rolling side to side with each stroke to make a narrower profile. To avoid wave resistance, Turetsky encourages swimmers to remove jerkiness in their stroke. (One of the other curious consequences of wave drag is that it punishes more shorter swimmers than their taller competitors. )
To achieve the reduced resistance technique Turkish swimmers train to improve their balance, locomotion and "feel" for the water. During training, the emphasis is on the quality of performance more than on mileage. His idea is that with constant repetition, precise skillful movements become second nature to them, like reflexes.
For this method to work properly, you need to pay attention to detail in training. "If you can't do it absolutely right, don't do it at all," Turetsky says. He would rather have his swimmers do a few moves right than lots of moves wrong. Turkish and his swimmers speak in terms of "muscle memory".
A lot of time is spent on proper technique, so by Olympic standards, Klim, Popov and the rest of Turetsky's squad have relatively leisurely training, although they still swim about 70 kilometers a week. To outsiders, his methods seem strange. An American coach, Bill Irwin, once told a journalist: “Popov performs long sets with meticulously accurate strokes and with invariably beautiful fluidity. For all three weeks, I did not see a single swim from him so that he looked tense.
<
p style="text-align: justify;"> Take it slow
Part of what we've seen is Turkish's "super slow float" method. Turetsky demonstrates this by walking around his office at an exaggeratedly slow pace. Moving very slowly, he must focus on the exact position of each muscle. Balance becomes necessary. “People become more unsteady when they move very slowly and they have to constantly shift their weight to stay in balance,” he says. The same applies to the pool, and when swimmers can move smoothly at a very slow speed, they can move more smoothly and at high speed.
Super-slow swimming also forces swimmers to focus on extending their arm as far as possible for maximum length on each stroke. And it improves the swimmer's ability to relax at higher speeds. When you are absolutely sure that your hands and feet will be in the right place at the right time, then during the race there is less frantic movement and less energy wasted. Relaxation is often overlooked, but the great American swimmer Johnny Weissmuller once said that “the greatest secret of freestyle swimming is to relax at top speed.” (Weissmuller is best remembered today for his Hollywood performances of Tarzan, but when Popov arrived, he was the only swimmer to win two gold medals in the 100-meter freestyle at two consecutive Olympics.) Turetsky elaborates: “Not all muscles fire at the same time. In the muscles, waves of contraction and relaxation pass simultaneously. The ability to relax muscles that are not being used conserves energy and prevents fatigue.
Slow speed training also helps the swimmer hone the most important intuitive "sense" of the water, to anticipate, control and manipulate its flow. Swimmers become mystics when they describe this ability, much like artists describe the "eye of God" when painting. For a swimmer, "feel", you know, is when you properly grab the water with your palm and drag your body forward with minimal resistance.
If super-slow swimming does not help develop this feeling, Turkish tries the opposite approach using his tow trainer. It pulls swimmers through the water at high speed so they get a heightened sense of what's going on when they position their arms and legs correctly. It's like sticking your hand out the window of a moving car, when your palm is held upright, you feel the wind drag it back. Rotate it 90 degrees and your hand will pass through the air like a knife.
Turetsky's methods are designed to optimize what he calls the "three Rs": stroke rate, relaxation, and rhythm. Rhythm is important for reducing jerkiness in the water. When a freestyle swimmer's arm enters the water, his or her body speeds up, but as it exits the water, the body slows down. Like a single-cylinder engine, this results in uneven progress. The more changes, the more energy is wasted.
To move at a constant speed, one arm must always enter the water while the other exits, so the movement is more like that of a two-cylinder engine, where one piston is moving while the other is recovering. To keep their arms moving in sync, Turetsky has his swimmers practice the "kayak maneuver" in which they stand by the pool with a double-bladed canoe paddle and perform an imaginary ride. Popov shows how during the swim, his one hand always remains opposite the other. Again, Turkish swimmers practice this way until the technique becomes second nature to them.
These unusual exercises and training methods seem to be paying off. Turkish swimmers don't use much energy to create waves. Further proving his success, a study by Sergei Kolmogorov, scientific director of the Russian team, showed that Popov's smooth technique allows him to consume 30 percent less energy than other swimmers moving at the same speed.
<
p style="text-align: justify;"> Turkish hopes to further improve his swimmers' technique. "I think Michael [Klim] will look better with time. He is still learning, still growing. I fight for beautiful technique,” he says. "Beauty and perfection are very close. "
Daniel Drollett is a freelance science writer in Australia on a Fulbright scholarship.
See also:
- Get to know more effective crawl swimming
- Freestyle stroke timing
- Swimming speed and stroke length
- "Swim Like a Fish" by John Wiedeler, Chapman & HaD (1993).
- "Sailing into the 21st century", Cecile Colvin, Human Kinetics (1992).
Translated by Svetlana Leshchenko
- Participate for FREE in the online training “Krol. Swimming is easy”, improve your swimming technique in a week…
- Download the book "How to learn to breathe easily in swimming" ...
- Training at the swimming school "It's easy to swim" ...
FISH SWIMMING MYSTERY | Science and Life
Wildlife often baffles researchers, presenting them with various "technical" riddles. One of them, over which more than one generation of scientists puzzles, is how many marine animals, fish and dolphins manage to move in dense water at speeds that are sometimes inaccessible even for flying in air. Swordfish, for example, swim at 130 km/h; tuna - 90km/h Calculations show that in order to overcome the resistance of water and gain such speed, the fish needs to develop the power of an automobile motor - about 100 horsepower. Living beings draw energy from oxidative processes. But fish are cold-blooded creatures, their temperature is not much higher than the temperature of water, in which oxygen, by the way, is dissolved in a very small amount. Such power is unattainable for them! It remains to assume only one thing: fish somehow "know how" to greatly reduce the resistance of water. The hypothesis explaining this phenomenon was put forward by the professor of the Institute of Theoretical and Applied Mechanics of the Siberian Branch of the Russian Academy of Sciences Vladimir Ivanovich Merkulov (Novosibirsk).
Diving record holder - swordfish.
During the flow of a viscous liquid and even gases (they also have viscosity), a so-called boundary layer is formed near the surface - a region where the flow velocity changes from zero on the wall to some constant value away from it.
The smaller the velocity gradient, the weaker the friction in the flow, the more stable the flow.
The region around the toroidal vortex can be divided into two parts: an external inviscid flow with zero resistance and an internal one with a slight velocity gradient and, consequently, extremely low viscous friction.
This is what a sea water motor might look like, assembled from alternating magnetic and electrical poles. The interaction of fields causes flows of a conducting fluid, similar to flows in a toroidal vortex.
Experiments have shown that even a wavy 'washboard' surface can slightly reduce hydrodynamic drag, but only in a certain range of speeds.
Science and Life // Illustrations
When water flows around a smooth ball, a boundary layer appears on its surface, which stretches for the length of the ball's radius.
‹
›
View full size
The past 20th century has to its credit great achievements in the field of hydrodynamics. Theoretical, computational and experimental methods make it possible to solve any scientific and practical problem of hydrodynamics. However, there is one problem that many scientific teams have been working on unsuccessfully and which has passed almost unchanged into the 21st century. This is the problem of reducing hydrodynamic resistance.
In order to correctly assess the potential opportunities in this direction, let's turn to the champion of scuba diving - the swordfish. An adult of this large and very strong fish grows up to four meters in length and gains weight up to half a ton. Her upper jaw is elongated into a long xiphoid process - the rostrum. Biologists believe this strange formation is a weapon used by swordfish to stun prey by breaking into schools of mackerel and tuna.
In the United States of America, swordfishing is the national sport. They catch swordfish on a spinning rod, and for the fisherman there is a great opportunity to instrumentally determine its speed.
According to publications, swordfish can reach speeds of up to 130 km/h. Ukrainian scientists made a swordfish model, hung it on a speedboat and determined the resistance of the model and the power required for movement. In terms of the speed and size of the fish, the model experiences a resistance of 4000 N (408 kgf) and requires a power of 100 hp for its movement. (73.6 kW)!
It is easy to understand that such parameters are unattainable for fish and, therefore, the laws of hydrodynamics allow movement with much less resistance than is realized in all our models. This means that it is quite possible to reduce resistance, and our attempts in this direction do not contradict physical laws.
In addition to the above experimental data, some theoretical considerations can be given that prove the possibility of a significant decrease in resistance.
According to Newton's law, tangential friction in a viscous fluid is equal to the product of viscosity and the velocity gradient (the gradient shows how fast some quantity changes when moving per unit distance). For a longitudinal flow around a plate, the velocity gradient is inversely proportional to the square root of the viscosity. Thus, the tangential friction in this case turns out to be proportional to the square root of the viscosity. At the same time, for the flow in the annular gap, the velocity gradient does not depend on the viscosity and the tangential friction is proportional to the first degree of viscosity. If we take into account that the dynamic viscosity of water is of the order of magnitude 10 -6 , then the tangential friction for the plate and the same surface in the annular gap will differ by a thousand times.
An example of an external flow with a small velocity gradient is the motion of a toroidal vortex along its own axis of symmetry. In calculations, the flow region is divided by a certain sphere into two parts: an external inviscid flow outside the sphere and an internal vortex flow inside the sphere.
At the interface, the tangential velocity of both flows is the same, so the external flow has zero resistance. The internal annular flow, due to its limitations, has a friction resistance proportional to the first degree of viscosity. It is this property that explains the amazing ability of the annular vortex to move quickly and far in the air. In order to use the amazing properties of the annular vortex for practical purposes, it is necessary to reproduce the flows in it on some body.
Any surface composed of streamlines can be considered as the surface of some body. Inside the annular vortex there are many surfaces that can be considered nested one into the other tori (in fact, it is a tightly coiled spiral). Placing a suitably shaped body inside the vortex will maintain an outward flow with zero resistance only if we compensate for the flow deceleration created by the body surface.
In sea water, the necessary forces can be created by constant electric and magnetic fields.
To do this, you need to assemble a structure in the form of a torus from alternating ring magnets and electrodes. Their poles create mutually perpendicular electric and magnetic fields, which will force the electrically conductive fluid to move around the surface of the torus, creating a body force that compensates for the deceleration of the flow.
As calculations have shown, with a magnetic field strength at the poles of one Tesla, achievable by using permanent magnets, for the movement of a torus with a diameter of 2 m at a speed of 10 m/s, an electric power of 300 W is required. This is a hundred times less than what is needed to tow a plate of equivalent area (despite the fact that the electrical efficiency with these parameters is only 6%).
Body force can only be created in an electrically conductive liquid. In fresh water and even more so in the air, this possibility is absent. Therefore, it is of interest to consider flows with a small gradient created due to boundary deformation according to the traveling wave law.
This formulation of the problem was first formulated by the author in the sixties. At the same time, under his leadership, a number of theoretical and experimental studies of vortex structures were carried out both on a dolphin, which acted as a prototype, and in laboratory conditions.
In contrast to the flow along a fixed boundary, when a boundary layer with a large velocity gradient is formed, the traveling wave rearranges the flow into a periodic structure with a small velocity gradient. For some values of the phase velocity and amplitude of the traveling wave, the total friction vanishes. Naturally, the question arises at what cost this result is achieved. Energy losses consist of two terms of different nature. The first is the viscous loss in the fluid. Since the gradient of the flow under consideration is small, these losses, which are proportional to the viscosity, turn out to be very small. The second term is the loss in the most elastic coating. With resonant vibrations of the material in the fluid flow, the main, and not small, energy must be pumped from the elastic form to the kinetic one. Another part of the energy is dissipated in the material. These losses can be compensated either by the energy of the flow, which will lead to a proportional increase in resistance, or by an external energy source.
Separately, there is the question of the mechanism and energy required for the initial formation of vortices. With a suitable choice of elastic parameters, it is possible to ensure that the traveling wave is excited in the bow part due to the energy of the external flow, and in the stern part the wave disappears, returning the energy to the flow.
Let us now turn again to the swordfish with which the story began.
Note that another good swimmer, the polar narwhal dolphin, has a similar shape and, as we assume, a similar drag reduction mechanism. An interesting detail: the upper left tooth of the male narwhal develops into a spirally twisted tusk up to three meters long, similar to the rostrum of a swordfish. Its purpose is unclear. Aren't they the secret to the fast and economical swimming of the swordfish and the liar?
Our hypothesis is that the rostrum and tusk serve as vortex generators.